After more than a decade’s work, the prospect of full quantum networks exploiting entangled photons is drawing closer. That’s thanks to the work of researchers like Hee Su Park from the Korea Research Institute of Standards and Science (KRISS).
From 2005 to 2010 he built up a quantum optics lab to develop measurement techniques for quantum information technologies. He wanted to find out to what extent optical fibres guiding multiple spatial modes can be used for quantum communications. Quantum networks encourage ‘collaboration by scientists in diverse fields, and surely inspire a lot of novel and interesting ideas,’ Park explained.
And, findings from scientists like Park are already suggesting ways in which quantum networks could be integrated with fibre networks. Experimental approaches previously used different wavelengths of light and different types of fibre to the predominantly single-mode, single core fibre infrastructure that today underlies the internet. But, new methods increase the chances that quantum networks might integrate with existing systems. It may be at least 20 years until that becomes a reality – but findings are already being published that put the process in motion.
For quantum networks, the key physical phenomenon to harness is entanglement, where properties of at least two different particles are correlated. In quantum computers, entanglement enables calculations that are extremely diffi cult for classical computers, in applications such as code-breaking and simulating chemical systems.
Spin city
Andrew Forbes from the University of the Witswatersrand in Johannesburg, South Africa, groups the ways quantum communication experiments entangle photons into two extremes. The most common approach exploits correlation between the polarisation, or spin angular momentum, of two photons. In 2019 researchers at the Institute for Quantum Optics and Quantum Information (IQOQI) in Innsbruck, Austria transported polarisation-entangled photons 50km through single-mode fibre at the most commonly used telecom wavelength, 1550nm. ‘That’s relatively easy to set up,’ he said. However, it entangles only two states, specifically opposite spin directions, which limits the amount of quantum information that photons can carry.
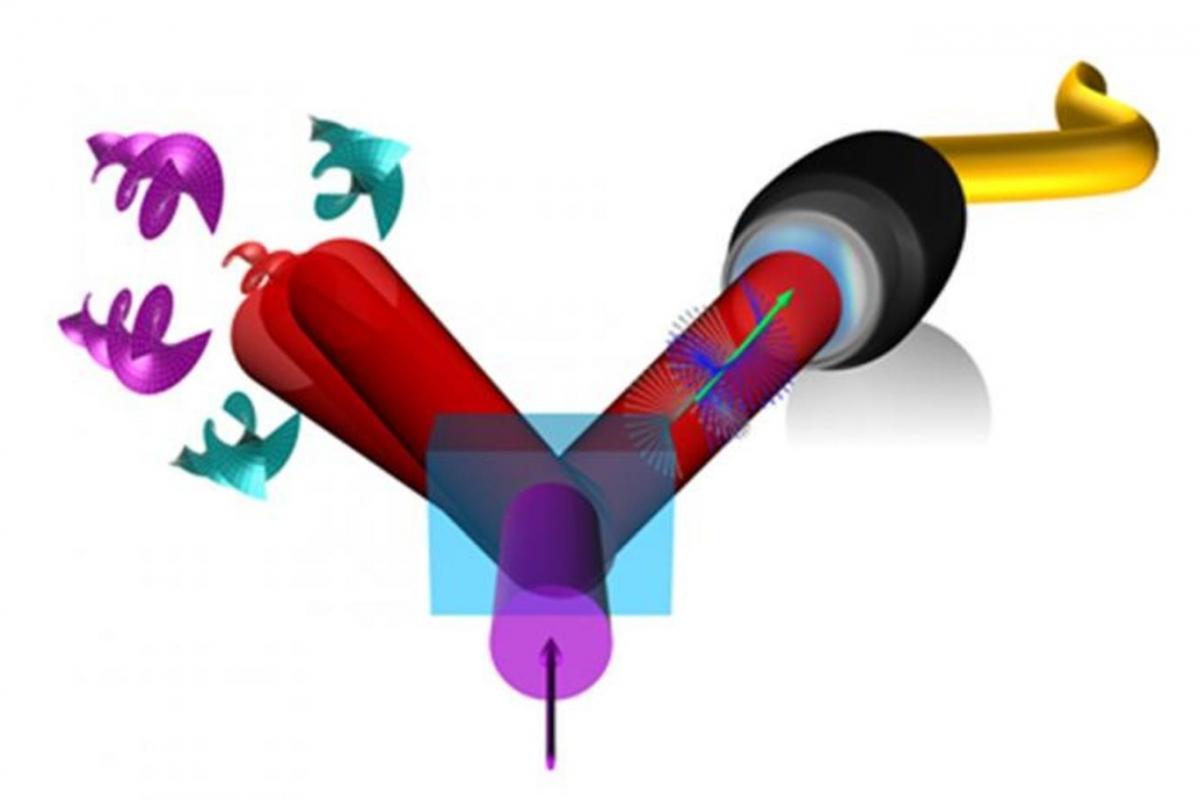
At the other extreme, observed Forbes, is spatial mode entanglement transport. This involves spatial light modulation, which integrates two light beams. It uses one beam to modulate the other’s spatial information, including its phase, polarisation state, intensity and propagation direction. Forbes noted that this can create ‘high-dimensional’ patt erns that potentially include an infinite number of entangled states. ‘You have now lots of information for every photon, but it’s extremely hard to get those patterns down fibre because patterns tend to couple into one another,’ he said.
Wolfgang Loeffler’s team, at Leiden University in the Netherlands, decided to study such spatial light modulation techniques of photon entanglement as far back as 2011, when they were relatively new. ‘Most work was done in theory and not much about fi bre transport of complex modes was known,’ Loeffler explained. ‘Nobody could answer the question “can we transport spatially quantum-entangled photons through an optical fibre?” So, we had to try this!’
Spatial delivery
The team exploited transverse mode components of light’s electromagnetic field that arise in order to ensure light remains within optical fibre. They were the first to transport spatially entangled photons through a hollow core photonic crystal optical fibre. They preserved entanglement over 30cm using light wavelengths around 826nm. Loeffler stressed that transporting high-dimensional spatial modes over longer distances is difficult.
‘It is already a challenge to phase-stabilise single spatial modes, which is required for specific quantum network types,’ he said. ‘This is even harder for many spatial modes, so I think that for quantum network applications, single-mode will be the transport of choice. Not even classical information is transported via long-distance multimode fi bres due to intermodal dispersion. But, who knows? If the advantages of high-dimensional quantum states weigh stronger than technical issues, the case may be different. High-dimensional quantum entanglement can be made also amongst spectral modes, not only spatial modes, so it can be sent through single-mode fibres.’
Park’s team also exploited spatial entanglement, having worked on linearly polarised (LP) modes in research published in 2012. ‘Photons guided by optical fi bres are in discrete modes having particular spatial field distribution in analogy with electrons residing in discrete energy levels in a potential well,’ he explained. ‘Each LP mode becomes a logical
quantum state of photons, and photons can be present generally in superposition states of those modes.’
Split the difference
Using this approach, the KRISS team split two entangled photons with wavelengths near 810nm and sent them down separate 40cm-long two-mode fibres. ‘To the best of my knowledge, we are the only group that has demonstrated propagation of two spatially entangled photons respectively through two different fibres,’ Park said.
In 2019, the KRISS researchers extended this approach into two 50cm-long multicore fibres to entangle four spatial modes. They used light with telecom-appropriate wavelengths around 1,550nm. ‘The two works used the same basic principles to generate spatial entanglement and numerically reconstruct quantum states from the set of measurement data,’ continued Park.
These studies show the feasibility, benefits and also the shortcomings of fibre transport of high-dimensional quantum states. ‘Such high-dimensional states, carrying more than one bit-per-photon, can increase the capacity limit of quantum communications imposed by the operation speeds of single-photon sources and detectors,’ said Park. ‘Our work also identifies the practical limitations of transmission distance due to non-idealities of the fibres and presents the methods to characterise those limiting factors.’
However, Park stressed how important high-dimensional entanglement could be in quantum networks. ‘By transferring the quantum state of entangled photons to the qubits of quantum computers, two physically separated quantum processors can be, in principle, connected by entanglement to build a distributed quantum computer,’ he explained.
‘High-dimensional encoding helps us to increase the amount of quantum links that can be generated by single photons. Of course there are a huge lot of technical challenges regarding connection between different types of qubits and long-distance transmission of photons.
On the right wavelength
Existing quantum computers are based on superconducting or ion-trap qubits. These oſten interact with microwave-range photons that have centimetre wavelengths, putting them far outside the telecom window. Park noted that there are theoretical proposals to inter-convert such photons to optical wavelengths. ‘I can only say that they are not impossible,’ he observed.
‘Quantum transduction to fibre-compatible frequencies is very challenging and an active research field,’ agreed Loeffler. ‘This is one reason why also considerable investments are done in the development of optical quantum computers, where this transduction would be much simpler and efficient.’
He therefore hopes that researchers will be able to improve systems to achieve photon coupling good enough in order to demonstrate nontrivial quantum networks in a few years. ‘Many exciting technologies will be developed on the side – this already is justifying the efforts for me,’ Loeffler said. ‘From a pure progress of research point of view, worldwide entangled quantum states would enable a number of very exciting new experiments, from precision metrology to tests of fundamental physics.’
The IQOQI team experiment using polarisation entangled photons has already shown what’s possible for transduction to telecom wavelengths. It collects 854nm photons from a trapped atomic ion qubit. To get the 50km range, the researchers converted these photons to 1550nm by combining them with 1902nm pump-laser photons in a polarisation.
Keep it simple
The work of Caspar van der Wal’s group at the University of Groningen is one of several teams developing semiconductor-based qubits that could simplify such transductions. Oſten the semiconductor is diamond and the qubits occupy nitrogen vacancy (NV) sites in their structure, explained Tom Bosma, a member of van der Wal’s team.
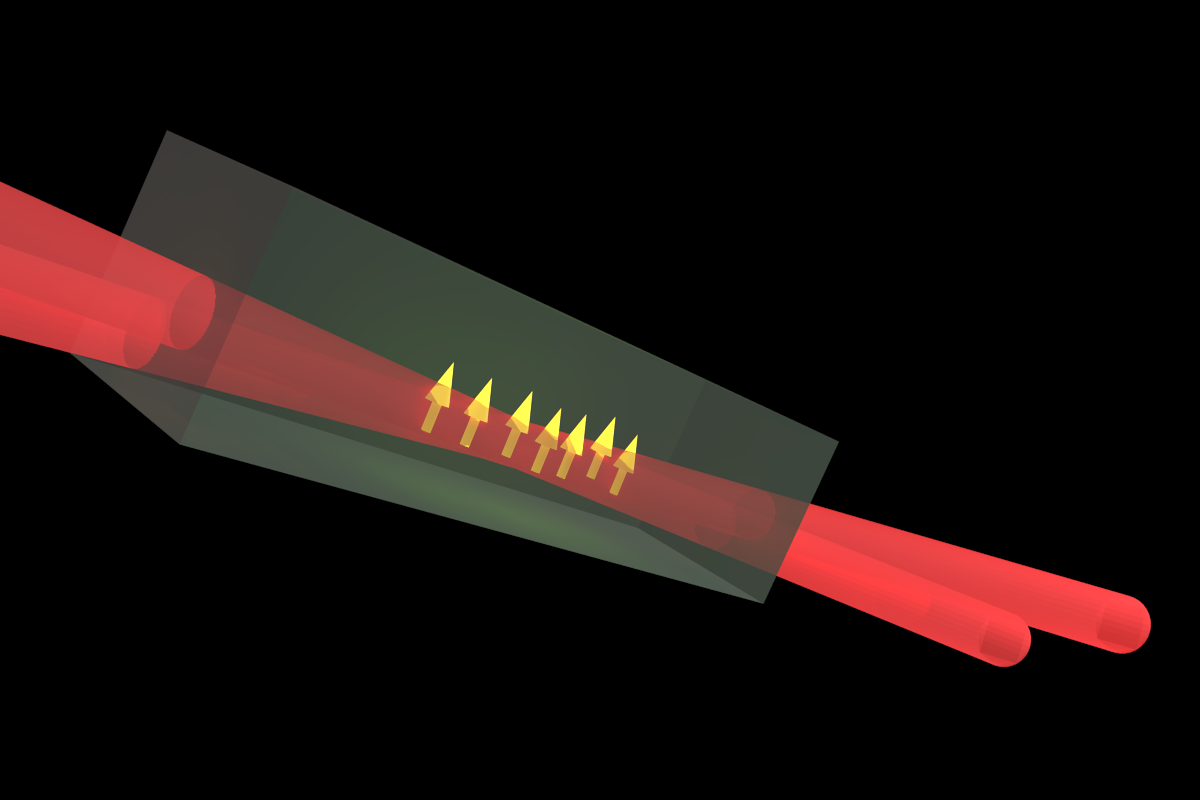
NV centres occur when a nitrogen atom substitutes for a carbon atom, carrying an extra electron whose spin can be used as a qubit. ‘Their spin lifetime and coherence times are sizeable even at room temperature,’ Bosma said. ‘It’s more scalable because you can basically make every defect act as a qubit throughout a very large piece of material. Then still, you need to entangle them with each other, which is going to be a challenge. But, one other thing with semiconductor qubits is that you can integrate them more with existing silicon electronics.’
Bosma noted that NV centres also produce photons with wavelengths around 600nm. While this is closer to telecom wavelengths than better-developed ion-trap and superconducting qubits, this wavelength has attenuation lengths in fibre below a kilometre. ‘You would lose your photons that carry the entanglement,’ Bosma said.
Silicon carbide (SiC)-based materials are similar, he added, but cheaper and easier to produce, and better suited to integration with existing telecom infrastructure. While it’s less well studied, researchers can form qubits where either silicon or carbon atoms are absent, or where one of both types of atom are missing immediately adjacent to each other. Most important for tuning the wavelength of a SiC-based qubit is the fact that it’s easier to dope than diamond, by intentionally adding metal impurities. ‘We’re looking into vanadium defects that actually emit at 1300nm telecom wavelengths,’ Bosma said. ‘And they have, it seems, promising spin properties.’
Link and repeat
Researchers at Delſt University in the Netherlands are already building quantum networks based on NV centres in diamonds, Bosma said. ‘Then, just like in normal fibre communications, at some distance you need to repeat the signal,’ he noted. ‘We think that silicon carbide might actually fit in as a quantum repeater, that you could in principle, emit at the right wavelength. You could do some entanglement swapping, and then repeat your entanglement through much larger distances.’
Such repeaters could enable quantum systems interacting ‘like the internet of today,’ Forbes suggested. The erbium-doped fibre amplifiers that serve as repeaters roughly every hundred kilometres in a classical fibre network ‘copy’ the beam to boost its signal. ‘That’s not allowed in the quantum world,’ Forbes said.
A leading potential approach that quantum repeaters might adopt would be to separate polarisation entangled photons, sending one to a quantum memory and the other to a Bell state measurement. This stores the entangled outcome of the measurement in the memory, prior to being sent from the memory down the next fibre link. In this way, the repeater
progressively entangles systems that have not interacted previously.
The Witwatersrand team, together with Scottish collaborators, were first to publish work on quantum repeaters for more complex, information-rich orbital angular momentum entanglement, Forbes claimed. Their all-optical approach uses an interference phenomenon from quantum optics called the Hong-Ou- Mandel effect to entangle photons that had not previously interacted. He is now working with collaborators on quantum repeaters that mix patterned spatial entanglement modes and polarisation entanglement. And now, as an off shoot of this work, Forbes and his colleagues have found ways to pass information-rich spatially entangled photons down conventional fibre.
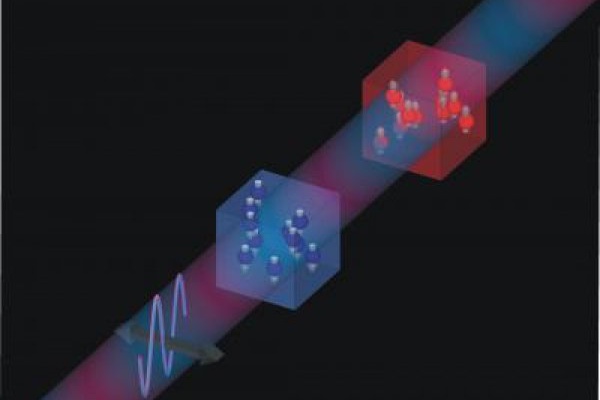
Measure for measure
‘We can make it look as if one photon is only entangled through polarisation and the other photon is only entangled through pattern,’ Forbes said. ‘We can take the photon that looks like it’s only polarisation entangled and send it down conventional fibre.’ Then, at the other end, a measurement extracts the spatial pattern information, he added. ‘In the quantum world, the photon doesn’t know about that until you make the measurement.’
Forbes stressed that his group’s aim is to use such findings to build a toolkit that helps push information capacities higher. That would build on cutting-edge polarisation entanglement studies, like the Nature paper from Jian-Wei Pan’s team at the University of Science and Technology of China, Hefei, China in February 2020.
The team established a link between two quantum memories comprising around 100 million laser trapped and cooled atoms over 11km of commercial optical fibre. Similarly, to the IQOQI team, they shiſt the 795nm photon output from the quantum memory using a 1,950nm pump laser to move it to the 1,342nm telecom O band. Forbes called these
achievements ‘crazily difficult experiments’, and used them as a measure for how soon quantum networks will be a reality. ‘How far is it from being commercially realisable? If you can get your work into Nature, it means that it’s 20 years away from being deployable.’